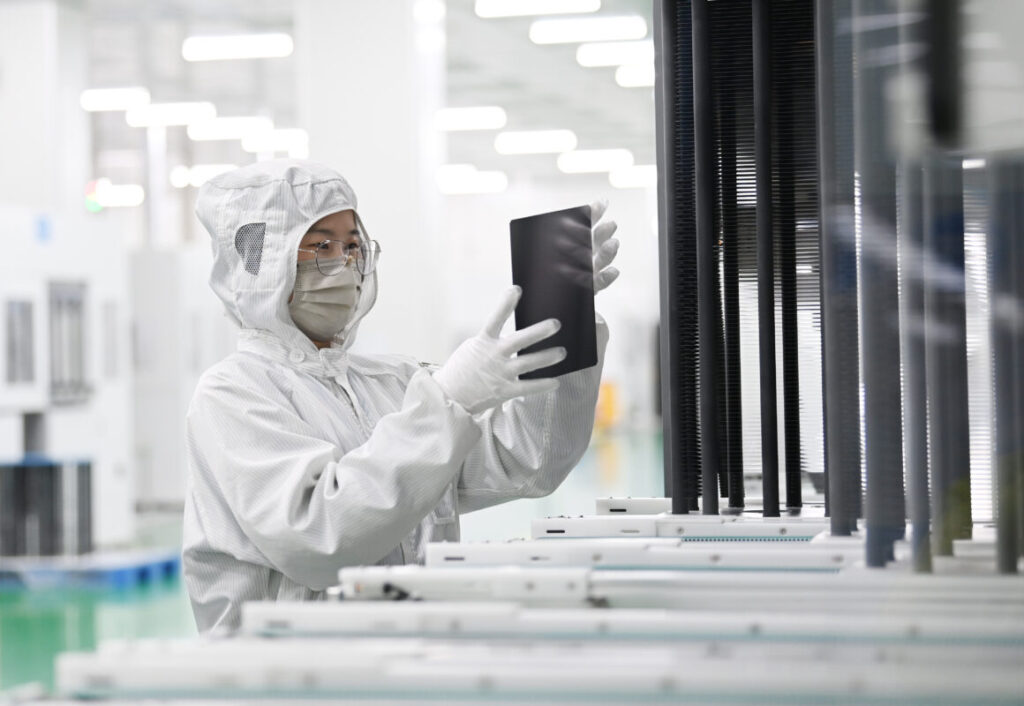
As TOPCon technology dominates the PV industry in 2024, concerns about ultraviolet-induced degradation have emerged in both academia and industry. Wenzhong Shen, Sheng Ma and Huanpei Huang present an overview of the phenomena, mechanisms and solutions of the UVID issue in TOPCon solar cells and modules, aiming to ensure the healthy development of the technology.
As a sustainable and environmentally friendly energy source, solar PV plays a central role in the transition from fossil fuels to a greener energy future. Continuous advancements in PV technology have not only improved energy conversion efficiency but have also brought attention to the reliability of these modules over long-term outdoor operations. Ensuring the durability of PV systems is crucial, as they are exposed to harsh environmental conditions that can negatively impact performance. At the core of any PV module is the solar cell, the essential component responsible for converting sunlight into electricity. However, in real-world applications, solar cells are exposed to a range of environmental stressors that challenge their stability and longevity.
Unlock unlimited access for 12 whole months of distinctive global analysis
Photovoltaics International is now included.
- Regular insight and analysis of the industry’s biggest developments
- In-depth interviews with the industry’s leading figures
- Unlimited digital access to the PV Tech Power journal catalogue
- Unlimited digital access to the Photovoltaics International journal catalogue
- Access to more than 1,000 technical papers
- Discounts on Solar Media’s portfolio of events, in-person and virtual
One of the most prominent stressors is sustained exposure to ultraviolet (UV) irradiation, which causes significant damage over time. Ultraviolet-induced degradation (UVID) refers to the phenomenon that the material properties and electrical properties of solar cells gradually decline after long-term exposure to UV irradiation. This degradation negatively impacts the solar cells’ photoelectric conversion efficiency, operational lifespan, and overall stability. UV irradiation, a high-energy component of the solar spectrum, is classified into three categories based on wavelength: UVA (320-400 nm), UVB (280-320 nm), and UVC (100-280 nm). Although UVC has the highest energy, the earth’s atmosphere absorbs most of it, leaving UVA and UVB, which can penetrate the atmosphere, as the primary contributors to material degradation.
The Renewable Energy Test Center (RETC) released its “Photovoltaic Module Index Report” in 2022, highlighting that certain emerging solar cell technologies, particularly n-type tunnel oxide passivated contact (n-TOPCon), appear to be vulnerable to UVID. The report presented concerning findings from UVID tests conducted on TOPCon modules, underscoring potential reliability issues. As shown in Figure 1, in 2022, researchers from the SLAC National Accelerator Laboratory and the National Renewable Energy Laboratory (NREL) recorded significant front and rear power losses in advanced solar cell technologies after conducting artificial accelerated UV irradiation tests [1].
By 2023, UVID testing standards became a major topic of discussion at the spring meeting of the IEC Photovoltaic Technology Expert Committee. In 2024, RETC’s PV index report further exacerbated concerns by revealing that 40% of tested photovoltaic module samples exhibited red-light warning signals, with some mass-produced and commercialised modules showing double-digit power losses. These findings suggest that certain modules may degrade by 10-16% within the first three years of operation, far exceeding the terms of module warranties and the power guarantees of photovoltaic systems. By this point, UVID had emerged as a critical topic within the solar industry, raising alarms about long-term reliability and prompting the need for more stringent testing protocols and technological improvements to mitigate the effects of UV irradiation.
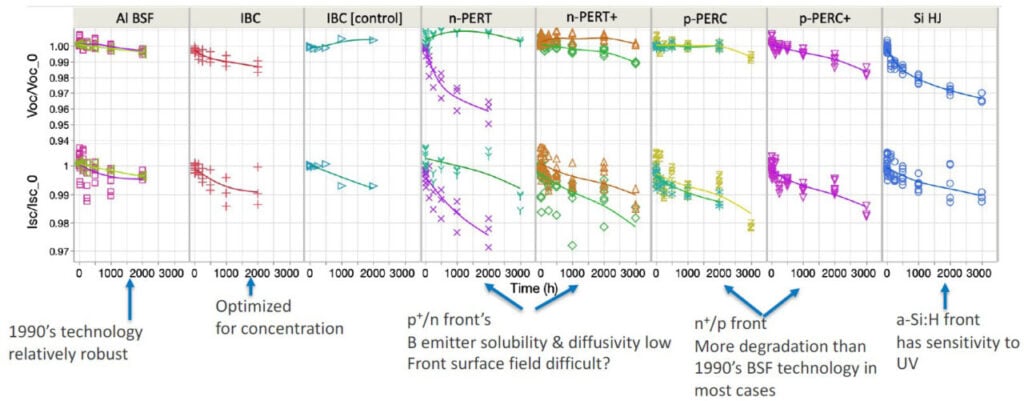
To effectively evaluate UVID in photovoltaic modules, it is essential to define specific test methods. In the IEC 61215-2 standard, the UVID test is characterised by UV irradiation within the spectral range of 280nm to 400nm, with the UVB component accounting for 3-10% of the total. The cumulative UV irradiation required for the test is 15kWh/m², compared to 60kWh/m² in IEC 61730, while maintaining the module temperature maintained at (60 ± 5) °C. In real-world conditions, the energy from UV irradiation constitutes approximately 5-8% of total solar radiation, and the 60kWh/m² of UV irradiation specified in the test corresponds to about 6 to 9 months of UV exposure in China.
The spectral similarity method is commonly used in laboratory UVID tests for photovoltaic modules. In this method, the UV spectrum of sunlight is simulated using specific light sources, such as xenon or metal halide lamps. Key parameters measured during UVID testing include UV light intensity, spectral distribution, temperature and humidity. The test conditions can be further customised based on specific application scenarios, ensuring that the test results are comprehensive and representative of the module’s expected performance in various environments. Through this approach, researchers can evaluate the extent of UVID and its potential impact on the longevity and efficiency of photovoltaic modules.
The mechanism of UVID for TOPCon modules
According to recent reports, the mechanism underlying UVID in PV modules is primarily linked to the properties and behaviour of the passivation layer. Different types of solar cells employ distinct passivation structures, leading to variations in the susceptibility to UVID varies across technologies. As early as the 6th International Silicon Photovoltaic Conference (Silicon PV 2016), ECN Solar introduced the concept of UVID, identifying that UV irradiation activates interface defect states, which increases the density of these defects, subsequently causing the deterioration of chemical passivation. Furthermore, UV irradiation can affect the fixed charge (Qf) at the passivation interface, leading to a decline in field-effect passivation.
For silicon nitride (SiNx) passivation layers, however, the UVID mechanism is more complex. The high energy of UV photons exceeds the bond energy of the Si-H bonds at the interface, resulting in the breaking of these bonds and the escape of hydrogen atoms during UV irradiation [2]. This loss of hydrogen degrades the passivation performance by increasing interface defects and reducing the overall effectiveness of surface passivation. In addition, studies indicate that UV irradiation causes the formation of suboxide at the SiNx/Si interface, further increasing the density of interface defects.
Another explanation for UVID degradation, specifically in SiNx passivation layers, is related to the deposition process. During plasma-enhanced chemical vapour deposition (PECVD) of SiNx, a damaged layer approximately 50 nm thick forms on the crystalline silicon side of the SiNx/Si interface [3]. This damaged region is initially passivated by hydrogen atoms, but UV irradiation accelerates the recombination rate in this layer, reducing carrier lifetime and negatively affecting the cell’s performance. Thus, the UVID mechanisms for SiNx passivated structures are multifaceted, involving both bond breakage and the formation of defects, which together degrade the photovoltaic cell’s efficiency over time.
The generation of hot carriers (electrons or holes) is another proposed mechanism contributing to UVID in PV modules [4]. When high-energy UV photons excite electrons, these electrons can be elevated beyond the valence band into the conduction band, creating hot carriers. Hot carriers possess high kinetic energy and strong mobility, allowing them to cross interface barriers in solar cells. In silicon-based cells, these hot carriers can acquire enough energy to damage the passivation layer, leading to an increase in interface state density. Certain materials, such as dry-oxidised silicon dioxide or polysilicon, offer greater resistance to hot carrier damage. Once hot carrier-induced damage occurs, it can often be repaired through annealing. Annealing at a temperature range of 250-300°C can restore the correct charge state of the passivation layers.
There is evidence to suggest that some interface defects generated by UV irradiation are hydrogen related, which explains why annealing in a hydrogen-containing atmosphere can completely restore these defects. In contrast, annealing in a nitrogen-containing atmosphere can only partially restore the damaged passivation layers. For AlOx passivation layers, under UV irradiation, the density of defect states at the AlOx/Si interface increases, leading to degradation in the chemical passivation effect.
Simultaneously, the fixed negative charge in the AlOx layer becomes more prominent, which enhances the field-effect passivation of the cell. This dual effect makes AlOx/SiNx passivation layers exhibit better UVID resistance compared to SiNx alone, as the field passivation provided by the AlOx compensates for the loss in chemical passivation. In summary, hot carrier generation under UV irradiation contributes to the breakdown of the passivation layer by increasing interface defects and facilitating degradation.
We have conducted UVID tests on both cells and modules, separately. Figure 2 illustrates the attenuation of UVID in TOPCon solar cells can vary significantly between different manufacturers. This variation is due to differences in the materials, passivation layer structures and fabrication processes employed by each manufacturer. In addition, due to the difference in the passivation layers between the front and rear sides of the cell, the UVID behaviour varies significantly.
The rear side of the cell exhibits superior resistance to UVID compared to its front. The front side of the TOPCon cell typically uses an AlOx/SiNx passivation layer, which relies primarily on the field passivation effect from the AlOx layer. However, the AlOx layer is particularly sensitive to UV irradiation, which results in a higher susceptibility to UVID on the front side.
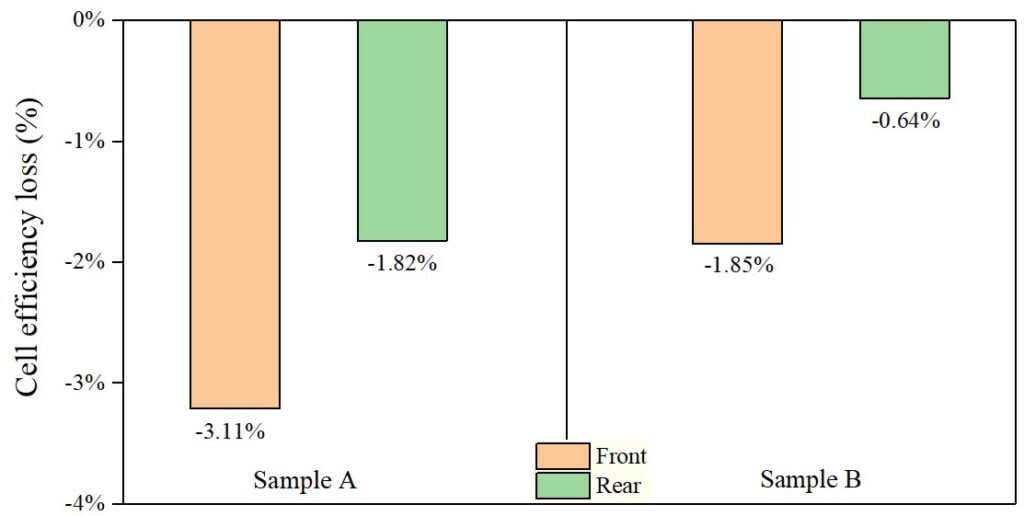
The interaction of UV light with AlOx can reduce its passivation efficiency, leading to power losses. In contrast, the rear side of the TOPCon cell is passivated with a SiOx/poly-Si/SiNx layer, which demonstrates better resistance to UVID. While the SiNx layer experiences some degradation under UV irradiation, the poly-Si layer plays a crucial role in preventing the damage caused by hot carriers generated under UV irradiation [5]. This is because the passivation performance of poly-Si is primarily based on its high doping concentration, making it less vulnerable to UV degradation.
Another significant factor affecting UVID performance is the variation in the optical properties of the SiNx layers on the front and rear sides. Typically, the rear SiNx layer is thicker than the front layer to enhance passivation performance, which increases UV photon absorption. This thickness difference leads to higher UV absorption by the rear-side SiNx, contributing to power losses. Additionally, under UV irradiation, the refractive index of the SiNx layer decreases, further exacerbating power loss.
This phenomenon is particularly pronounced in thin films with a high refractive index [6]. The concentration of hydrogen atoms in the SiNx layer also plays a crucial role in UVID. Changes in the refractive index of the SiNx layer under UV irradiation may also correlate with changes in hydrogen atom concentration.
This relationship between hydrogen concentration and UVID remains a topic for further study to better understand how UVID can be minimised in TOPCon cells. In summary, the UVID performance of TOPCon cells is influenced by the passivation structure. While the AlOx/SiNx film on the front is more sensitive to UV, leading to higher UVID, the poly-Si layer on the rear provides better resistance. The optical properties of the SiNx layers, particularly the refractive index and hydrogen concentration, are key factors in UVID behaviour and warrant further research for improving UVID resilience in TOPCon cells.
Potential UVID mitigation solutions
After understanding the degradation mechanism associated with UVID, we can develop effective strategies, which will vary depending on the stage of the issue. In the short term, addressing UVID can be achieved through the optimisation of the passivation structure and the use of appropriate packaging materials. However, it is important to note that the reliability of new material combinations and cell structures will require time for thorough verification.
For a long-term solution, we should focus on developing innovative materials and exploring new technologies, including advanced passivation techniques. By adopting these approaches, we aim to improve the overall performance and reliability of TOPCon modules, ensuring they maintain their efficiency and longevity in various environmental conditions. The following content will introduce the current strategies for solving UVID issues of industrial TOPCon.
First, from the perspective of cell technology, the impact of UVID can be effectively mitigated through the optimisation of the passivation film. One effective approach is to increase the refractive index of the SiNx film. A higher refractive index of SiNx can significantly enhance UVID performance because it reduces the penetration of UV photons at the SiNx/Si interface.
This reduction in UV photon interaction leads to less damage to the silicon substrate, thereby decreasing UVID. As displayed in Figure 3, when the refractive index of SiNx increases from 2.07 to 2.14, the corresponding cell efficiency loss drops from 2.54 to 1.95.
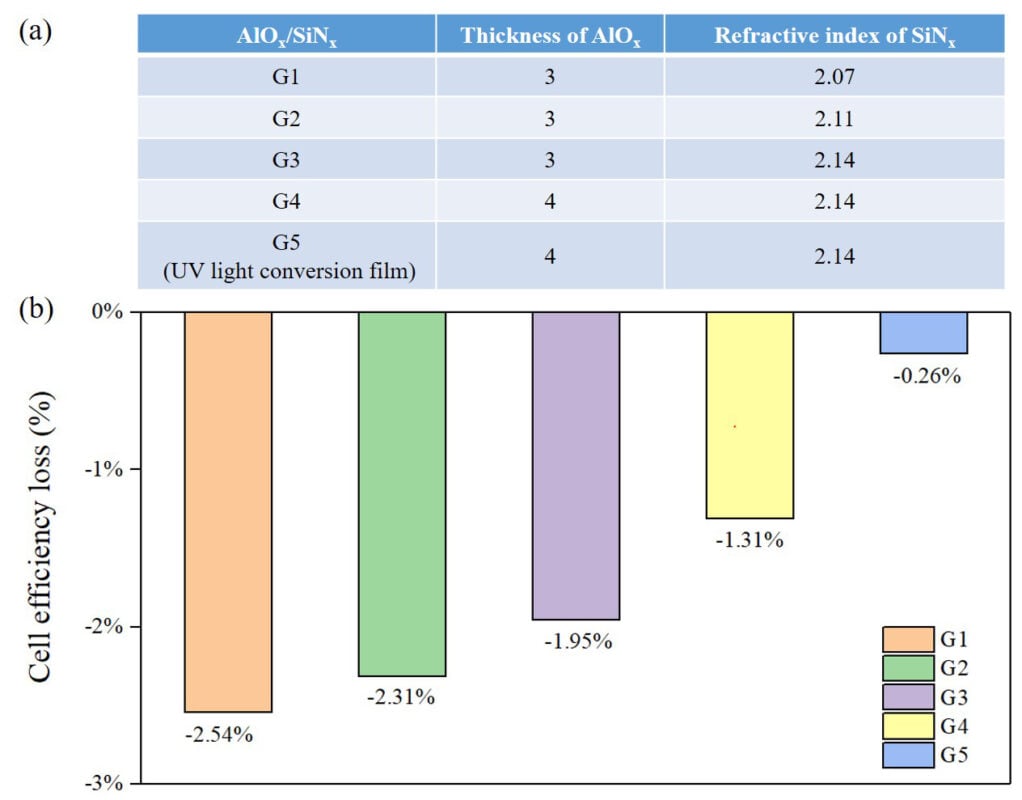
However, it’s important to balance this enhancement, as increasing the refractive index of SiNx too much can result in optical absorption losses. Therefore, the refractive index of SiNx must be optimised rather than maximised. Furthermore, it has been demonstrated that cells utilising an AlOx/SiNx film layer exhibit better UVID performance compared to those with a SiNx structure. This improvement is attributed to enhanced passivation due to the increased density of fixed negative charges in the AlOx layer under UV irradiation. In industrial TOPCon cells, the front side typically employs an AlOx/SiNx film. Many mainstream manufacturers enhance UVID performance by increasing the thickness of the AlOx film as seen in Figure 3.
This is effective because, within a certain thickness range, thicker AlOx films can improve the passivation performance and offset the degradation caused by UVID. Additionally, some manufacturers adopt a strategy of increasing the annealing temperature of the AlOx layer. This approach is beneficial as it results in an increase in the thickness of SiOx at the AlOx/Si interface [7]. The thicker SiOx layer can reduce the interfacial state density of the film, thereby improving chemical passivation and further enhancing the overall UVID resistance. Overall, these strategies highlight the importance of material optimisation and process adjustments in improving the resilience of solar cells against UVID.
From the perspective of packaging materials, reducing the influence of UVID can be achieved by utilising specialised materials that minimise UV light transmittance. For instance, cerium-containing glass and custom anti-reflective coatings have been considered for this purpose [8]. However, these options have not yet been widely adopted due to their high production costs and challenges related to recyclability. Another approach to mitigating UVID is the use of UV-cut films. While these films can effectively reduce UV irradiation, they can also block certain beneficial UV light wavelengths, potentially leading to a loss in overall power generation.
To address this drawback, UV light conversion films have gained attention [9]. These films function by absorbing UV light from sunlight and converting it into more favourable wavelengths, such as visible light, thereby enhancing the power generation efficiency of PV modules. Research has shown that light conversion films are effective in inhibiting UVID in heterojunction modules and are being increasingly adopted in industry. However, there have been no reports on the use of light conversion film for anti-UVID for TOPCon cells and modules.
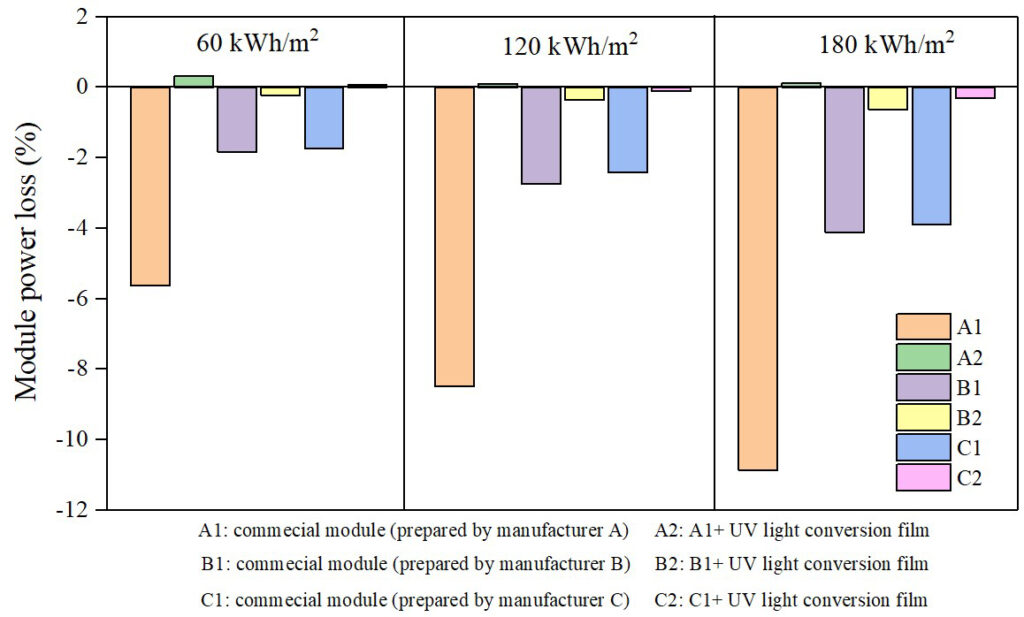
As seen in Figure 3, after the addition of light conversion film on G4, the value of UVID (G5) drops from 1.31% to 0.26%. To further illustrate this issue, we performed validation on the modules prepared by different manufacturers as seen in Figure 4. This result is obtained through a third-party certification agency, and the commercial modules are sourced from mainstream top-tier manufacturers in China. The variation in UVID across modules from different manufacturers, as observed in Figure 4 aligns with differences in cell designs employed by each company. Each manufacturer’s design choices, including materials, passivation techniques, and cell architectures—contribute uniquely to the module’s resistance or susceptibility to UV degradation. These design variations directly influence how each component handles UV irradiation, resulting in significant differences in UVID performance.
Additionally, incorporating a light conversion film during the packaging process has proven to be an effective method for reducing UVID. Light conversion films can work by converting harmful UV wavelengths into longer, less damaging wavelengths before they reach the cell, thereby mitigating the degradation effect of UV exposure on the cell materials and passivation layers. This approach enhances the overall stability and lifespan of photovoltaic modules by decreasing the rate at which UV irradiation negatively impacts the cells.
Conclusion
UV irradiation is a significant factor contributing to the performance degradation of PV modules, particularly observed in recent developments with TOPCon modules. UV irradiation can reduce the integrity of the passivation layers, which are critical for minimising recombination losses, ultimately affecting the efficiency of the solar cells. The values of UVID in TOPCon cells and modules can vary significantly between different manufacturers due to differences in the materials, passivation layer structures, and fabrication processes.
To address these challenges, at the present stage, it can be solved by the optimisation of the passivation structure and the use of appropriate packaging materials. Our experimental results show that the cell design can reduce UVID to a certain extent, and using light conversion film during packaging can control UVID at a lower level. In the long term, there is a need for the development of comprehensive testing standards and evaluation methods that can reliably assess the long-term stability and performance of different PV technologies under UV irradiation.
Creating a large database that compiles stability data from various photovoltaic technologies will facilitate a better understanding of their long-term performance in real-world conditions. Sharing this data among researchers and manufacturers can drive improvements across the industry. Building more robust models to analyse UVID mechanisms will provide deeper insights into how different materials and technologies react to UV irradiation. This understanding can inform future efforts and guide the design of more resilient photovoltaic systems. By implementing these measures, the photovoltaic industry can better mitigate the adverse effects of UV irradiation, ultimately promoting higher-quality products.
References
- P. Hacke, K. Hurst, D.C. Miller, et al. “Value proposition of UV absorbers in PV module encapsulation”, presented at the 38th Edition of the European Photovoltaic Solar Energy Conference and Exhibition (EU PVSEC), 6-10 September 2021.
- H.R. Ye, S.L. Huang, C. Qian. “Short wavelength photons destroying Si-H bonds and its influence on high-efficiency silicon solar cells and modules”, Sol. RRL, 2023, 7, 2300334.
- T. Kamioka, D. Takai, T. Tachibana, et al. “Plasma damage effect on ultraviolet-induced degradation of PECVD SiNx: H passivation”, in 2015 IEEE 42nd Photovoltaic Specialist Conference (PVSC), 2015, pp 1-3.
- P.J. Verlinden, R.M. Swanson, R.A. Crane. “High-efficiency silicon point contact solar cells for concentrator and high-value onesun applications”, in 12th European Photovoltaic Solar Energy Conference and Exhibition, 1994, 1477-1480.
- P.E. Gruenbaum, J.Y. Gan, R.M. Swanson. “Use of ultrathin oxides and thin polycrystalline silicon films for stable high-efficiency silicon solar cells”. Appl Phys Lett, 1991, 58(9), 945-947.
- R. Witteck, B.V. Wolf, H.S. Huxel, et al. UV-induced degradation of PERC solar modules with UV transparent encapsulation materials, Prog Photovoltaics Res Appl, 2017, 25(6), 409-416.
- C.H. Hsu, C.W. Huang, J.M. Lai, et al. “Effect of oxygen annealing on spatial atomic layer deposited aluminum oxide/silicon interface and on passivated emitter and rear contact solar cell performance”. Thin Solid Films, 2018, 660, 920-925.
- M.D. Kempe, T. Moricone, M. Kilkenny. “Effects of cerium removal from glass on photovoltaic module performance and stability”, in Proceedings of SPIE, 2009, 74120Q.
- CHOSHU INDUSTRY CO., LTD, Chromophores with enhanced solar light harvesting efficiency, high fluorescence, and photostability, Japanese Patent No. JP2012211071.
Authors
Prof. Wenzhong Shen received a PhD. degree in semiconductor physics and semiconductor devices from Shanghai Institute of Technical Physics, Chinese Academy of Sciences, China, in 1995. Since 1999, Dr. Shen has been with Shanghai Jiao Tong University, China, as a full professor in the School of Physics and Astronomy. Since 2007, he has been the director of the Institute of Solar Energy at Shanghai Jiao Tong University. His main research interests are in the fields of silicon solar cells, perovskite solar cells and perovskite/silicon tandem solar cells, as well as semiconductor quantum electronic devices.
Dr. Sheng Ma is a researcher with extensive expertise in the field of photovoltaic technology, specifically focusing on crystalline silicon solar cells and crystalline silicon/perovskite tandem solar cells. He obtained his PhD in Materials Science and Engineering from Shanghai Jiao Tong University, where he later continued his career as a full-time researcher. Before transitioning to academia, he accumulated significant industrial experience by working on the research and development of solar silver paste, solar cells and solar cell equipment in several top-tier companies in the photovoltaic industry.
Huanpei Huang received her B.S. degree in Shanghai Jiao Tong University in 2021. Currently, she is a PhD candidate at the School of Physics and Astronomy at Shanghai Jiao Tong University, focusing on the mechanistic research of solar cells. She has participated in multiple national projects related to photovoltaic cells. Her work targets the demands of large-scale photovoltaic power generation to continuously improve the efficiency and reduce the cost of crystalline silicon cells, advancing the design principles of high-efficiency solar cell structures and providing direction for cell manufacturing processes.