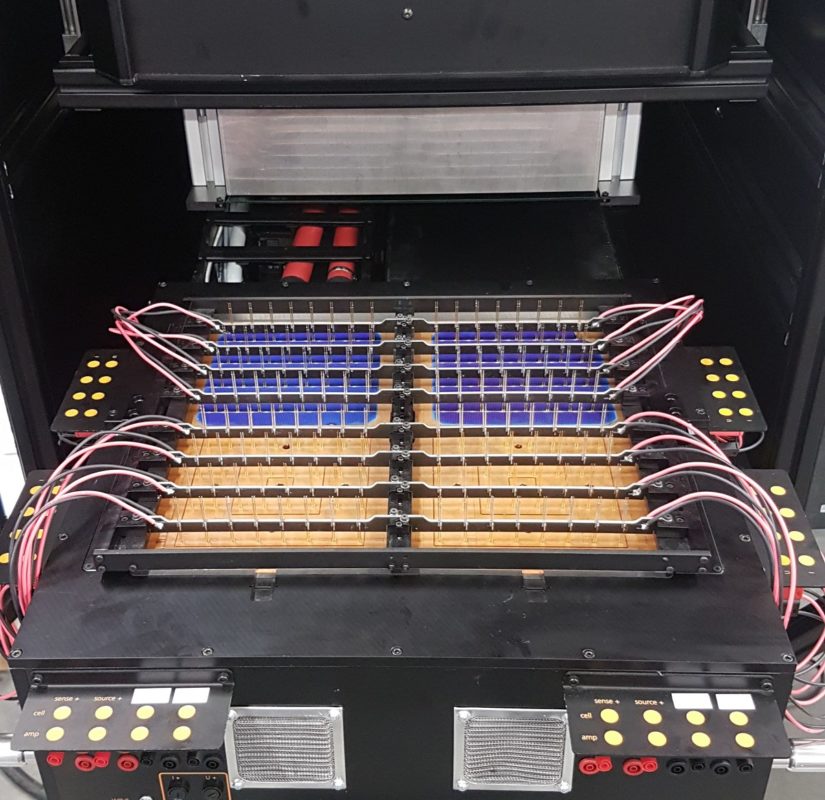
In a PVEL survey of 2018, light-induced degradation (LID, LeTID) was identified as the defect that causes the greatest concern among investors implying severe financial risks [1]. One reason is that the defect is still relatively new and not entirely understood due to its complexity. To reduce these risks, the LeTID Norm consortium is working on a standard to test the LeTID sensitivity. To this end, the consortium brings together the experience of research cell manufacturers, research institutes, test facilities and PV power plant operators. Thus, the proposed test standard is based on a better understanding of the defect that is causing LeTID combined with practical applicability of the test procedure.
Unlock unlimited access for 12 whole months of distinctive global analysis
Photovoltaics International is now included.
- Regular insight and analysis of the industry’s biggest developments
- In-depth interviews with the industry’s leading figures
- Unlimited digital access to the PV Tech Power journal catalogue
- Unlimited digital access to the Photovoltaics International journal catalogue
- Access to more than 1,000 technical papers
- Discounts on Solar Media’s portfolio of events, in-person and virtual
Light-induced degradation – the current scientific knowledge
The phenomenon of illumination leading to a loss of solar cell efficiency has been under investigation for more than 40 years. Several mechanisms causing such a degradation have been studied, including the activation of boron-oxygen-defects (BO), the dissociation of iron-boron-pairs (FeB), the degradation due to copper (Cu-LID), sponge-LID, and light and elevated temperature-induced degradation (LeTID). It is well known that all these defects are activated by charge carrier injection i.e. by illumination or current injection equivalently [2].
While most of these mechanisms are activated within minutes (FeB) or days (BO, Cu-LID and sponge-LID) during operation, it takes years until the LeTID degradation reaches its maximum [3]. Due to the significantly different timescales it is relevant to determine LeTID apart from the other LID mechanisms to estimate the overall losses during operation. A separation of LeTID is feasible as this degradation can only be observed above 50-60°C implying testing times of the order of weeks. Quite generally, the kinetics strongly accelerate with increasing temperature [2]. However, high temperatures over 75°C reduce the degradation extent, since the regeneration which occurs subsequently to the degradation is even more accelerated (see Figure 1). At a relatively low temperature of 25°C, a degraded cell exhibits a recovery of the degraded cell parameters under illumination. This recovery differentiates from the regeneration observed at elevated temperature, as it results in an instable state, which degrades again at an elevated temperature treatment [4].
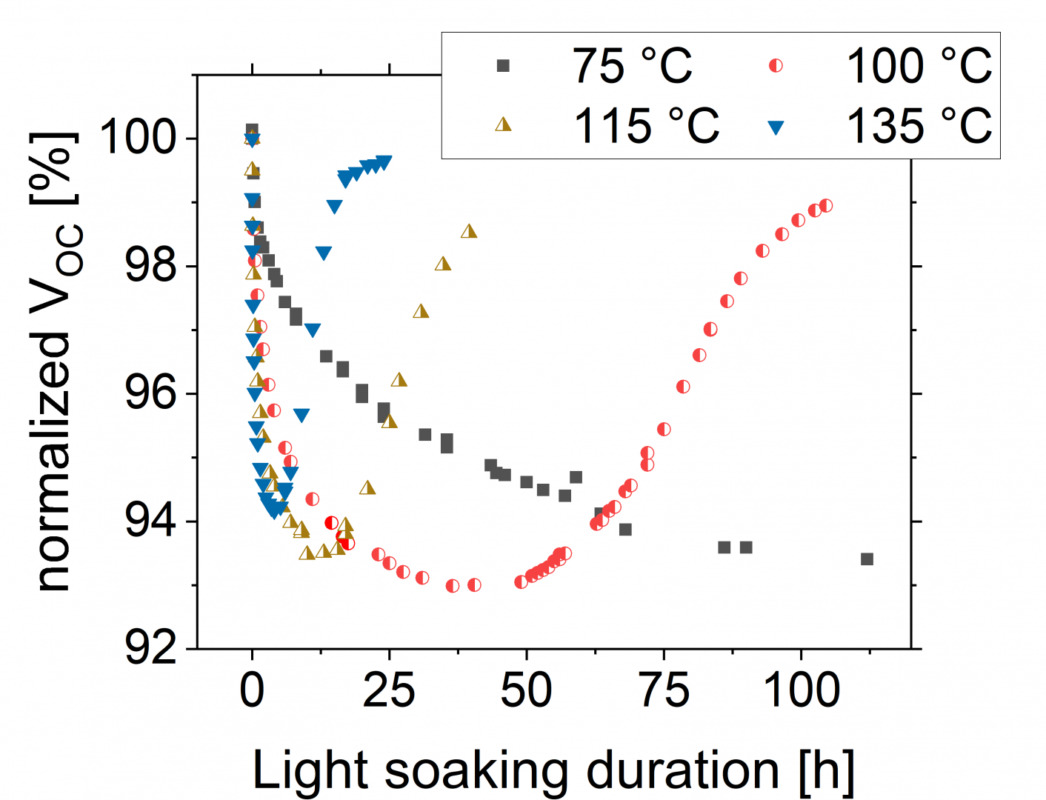
Investigations have also shown that the cell process strongly affects the degradation. The higher the temperature of the firing process step (the last high temperature step in cell manufacturing), the stronger the degradation [5]. Slower cooling rates after reaching the peak temperature during firing step can reduce LeTID [6]. Furthermore, pre-annealing before the firing step or post-annealing after the firing step can reduce LeTID [7]. It was also shown that thinner wafers [8] and gettering steps for metallic impurities reduce LeTID [9]. During the last years, several publications showed that a high hydrogen content introduced into the silicon from the silicon nitride passivation layer of a PERC cell leads to faster and stronger LeTID [10,11].
At the moment, there is no common model for the cause and description of LeTID. Due to the strong influence of hydrogen on LeTID the UNSW has presented a “three-bucket/four-state model”, which assumes that hydrogen is the only LeTID causal agent [12]. Schmidt et al. assume that 3d transition metal impurities are the main causal agent. In this model, the assumed state after firing is that the interstitial metal impurities are paired with hydrogen atoms and are assumed as recombination inactive [13].
Within the LeTID Norm project a model has been developed assuming that 3d transition metal impurities dissolving from metal-silicon-precipitates paired with hydrogen cause the degradation (see Figure 2). In this model, the well-known property of Co, Ni and Cu (all common impurities in PV wafers and cells in typical concentrations up to or more than 1013 cm-3) of the formation of small metastable platelets of the type MSi2 even after the fastest cooling to room temperature [14] are used to explain the low recombination activity after firing in spite of the presence of the metal impurities. This model covers all currently known facts about LeTID. Further investigations will be necessary to confirm or disprove these three models.
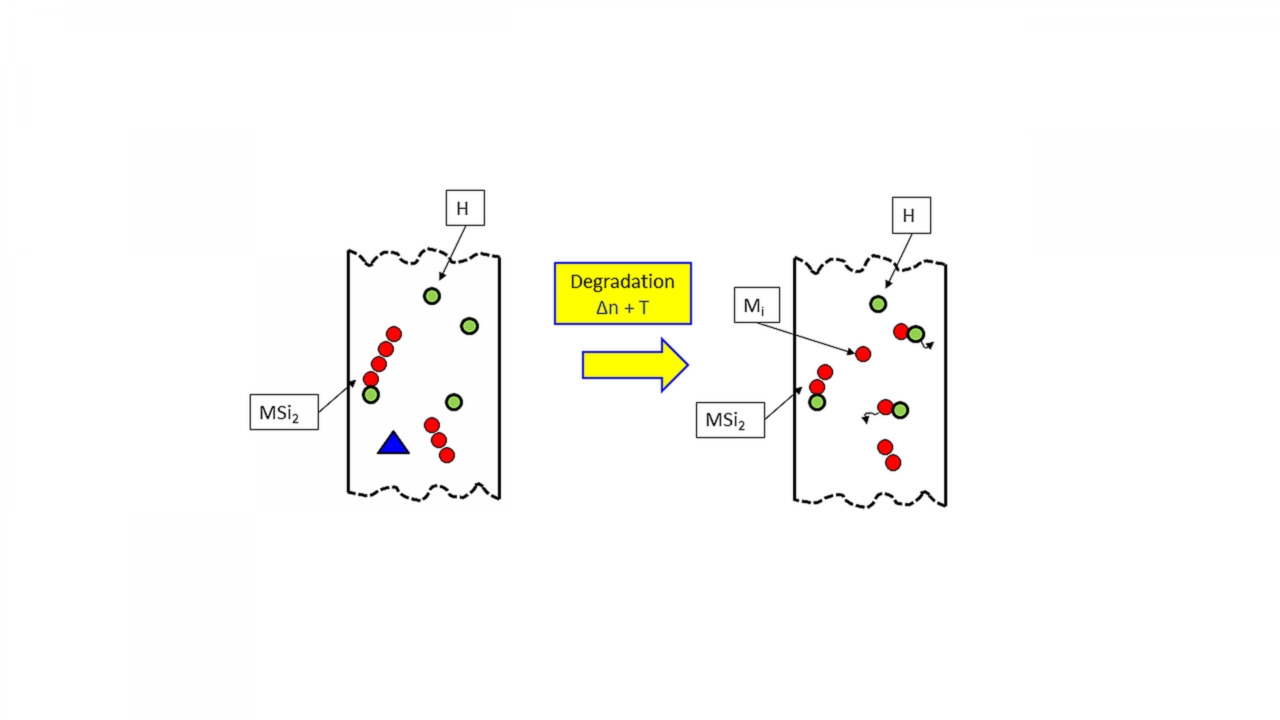
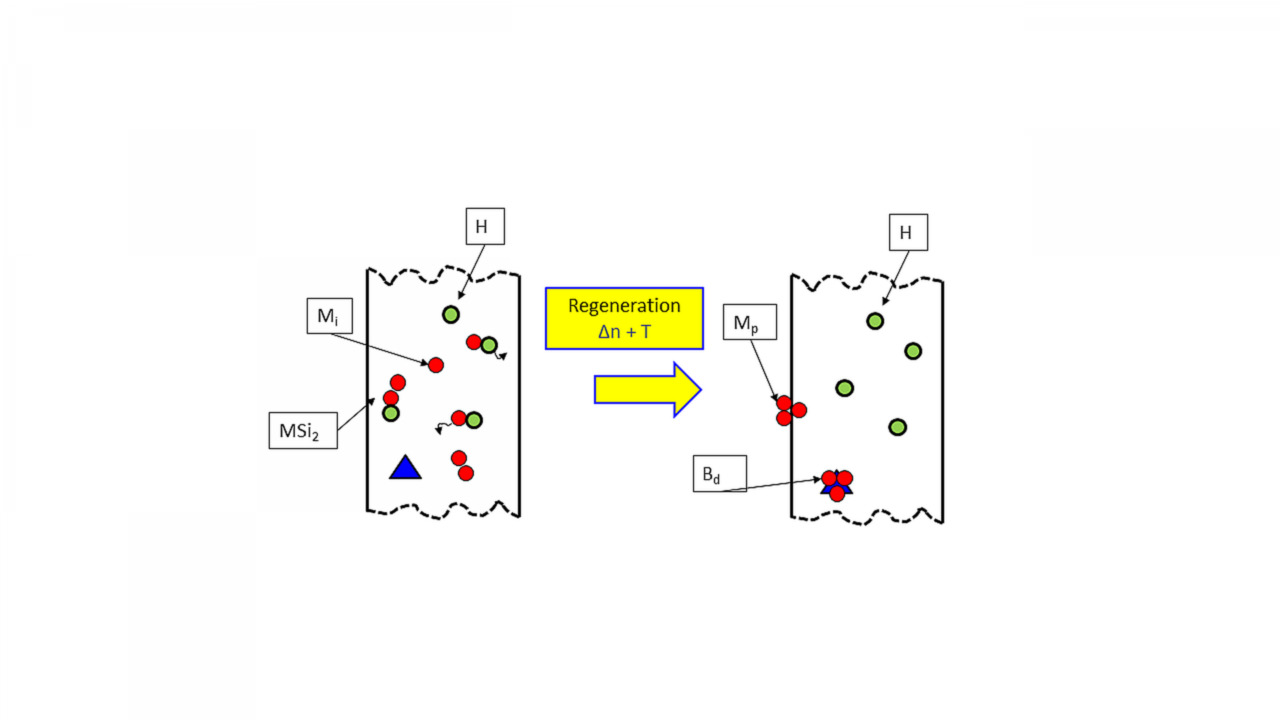
This is an extract of a technical paper first published in Volume 23 of PV Tech Power. The full article can be read here, or in the full digital copy of PV Tech Power 23, which can be downloaded for free here
Citations
[1] PVEL survey, 2018.
[2] F. Kersten et al. 2015 “Degradation of multicrystalline silicon solar cells and modules after illumination at elevated temperature”, Solar Energy Materials and Solar Cells;142:83-86.
[3] F. Kersten et al. 2017 “System performance loss due to LeTID”, Energy Procedia, Volume 124, 540-546.
[4] F. Kersten et al. 2015 “New light induced volume degra-dation effect of mc-Si solar cells and modules”, Proceedings of the 31st EU PVSEC.
[5] D. Bredemeier et al. 2016, „Lifetime degradation and regeneration in multicrystalline silicon under illumination at elevated temperature”, AIP Advances 6, 035119, 2016.
[6] R. Eberle et al. 2016, „Impact of the firing temperature profile on light induced degradation of multicrystalline silicon”, Physica status solidi – rapid research letters 10, 861-865.
[7] T.H. Fung, C.E. Chan, B.J. Hallam, D.N.R. Payne, M.A. Abbot and S.R. Wenham 2017 “Impact of annealing on the formation and mitigation of carrier induced defects in multi-crystalline silicon”, Energy Procedia Vol. 124, 726–733
[8] D. Bredemeier, D.C. Walter, and J. Schmidt 2018, “Possible Candidates for Impurities in mc-Si Wafers Responsible for Light-Induced Lifetime Degradation and Regeneration”, Sol. RRL 2, 1700159.
[9] D. Bredemeier, D.C. Walter, J. Schmidt 2018, Possible Candidates for Impurities in mc-Si Wafers Responsible for Light-Induced Lifetime Degradation and Regeneration, Sol.RRL. 2 1700159
[10] C. Vargas et al. 2018, “Carrier-Induced Degradation in Multicrystalline Silicon: Dependence on the Silicon Nitride Passivation Layer and Hydrogen Released During Firing”,IEEE JOURNAL OF PHOTOVOLTAICS, VOL. 8, NO. 2.
[11] U. Varshney et al. 2018, “Influence of dielectric passivation layer thickness on LeTID in multicrystalline silicon”, 7th WCPEC, Waikoloa, Hawaii.
[12] A. Wenham et al. 2018, “Hydrogen-Induced Degradation”, 7th WCPEC, Waikoloa, Hawaii.
[13] J. Schmidt, D. Bredemeier, and D.C. Walter 2019, „On the Defect Physics Behind Light and Elevated Temperature-Induced Degradation (LeTID) of Multicrystalline Silicon Solar Cells”, IEEE JOURNAL OF PHOTOVOLTAICS, vol. 9, no. 6, 2019.
[14] W Bergholz 1981, “On the diffusion of Co in Si and its applicability tothe Si intrinsic defect problem”, J. Phys. D: Appl. Phys. 14 1099 and J. Utzig 1988 “Properties of cobalt in FZ and CZ silicon studied by Mössbauer spectroscopy”, Journal of Applied Physics 64, 3629